Microgrids
Understanding the impact and integration within a complex energy environment
As the world surges towards decarbonization and decentralization, microgrids are standing out as a potential solution to some of the challenges facing everyone from governmental and regulatory authorities, to energy suppliers, grid operators and customers. In fact, microgrids are considered to be a secure, affordable, sustainable and low carbon option in many specific localized applications because by their nature, microgrids incorporate three key components; Generation, Storage and Demand all within a bounded and controlled network that may or may not be connected to the grid.
By their nature, microgrids support energy self-reliance and enable resilience against severe weather events, incorporate multiple distributed energy resources either traditional or renewable, and allow local communities to increasingly take control of their energy future.
For decision makers and planners in today’s energy sector, the energy revolution will include microgrids as a solution to deal with many economic and environmental factors, technological breakthroughs and legislation changes.
So, what are the trade-offs between several key priorities, such as cost effectiveness, supply resiliency, efficiency, decarbonization and commercial opportunity? Detailed technical and economic analysis are required as part of the scoping, design planning and operational maintenance of such systems. Though PLEXOS has typically been applied to the study of larger national or continental scale energy systems to date, it has more than enough modeling flexibility to be applied to systems at any scale, large or small.
We outline some of the typical characteristics of microgrids, list some example projects from around the world, and discusses the detailed technical and operational characteristics that can be integrated within the state of the art PLEXOS modeling tool to aid local energy system decision making and analytics.
“The Clean Energy for EU Islands Initiative aims at accelerating clean energy transition by helping islands reduce their dependency on energy imports and making better use of locally available renewable energy sources. It will also promote modern and innovative energy systems and help islanders reduce greenhouse gas emissions.”
- EUROPEAN COMMISSION
What Technologies make up Microgrids?
Technologies
There are few ‘typical structure’ definitions of a microgrid, as they can be applicable with a range of constituent technologies, energy vectors and design topologies.
Some of the possible components are listed in Figure 1 and Figure 2 on the following page. Common characteristics of a microgrid include that they are typically small in scale with peak power demand, e.g. ranging from tens of KWs up to tens of MWs, that they are usually able to operate independently from the main power grid or in synchronization with it, and that they generally have a rather small/localized geographical/spatial footprint.
They can incorporate multiple Distributed Energy Resources (DERs) that usually feed electricity as the main energy supply component, but can also incorporate links to gas networks, thermal energy and water supply elements. There can also be tangential connections to the transport sector, via electric or even hydrogen vehicles.
Distribution
Where available, a Point of Common Coupling (PCC) is used to provide the microgrid with connectivity to a larger scale main grid. When the PCC is temporarily or permanently unavailable, they become isolated microgrids, which is the default setup in the case of geographically remote sites. PCC connectivity is managed through either conventional AC switchgear in conjunction with power electronic converter controls.
Based on the characteristics of the electricity distribution system, microgrids can be classified into AC, DC or hybrid microgrids (those that combine elements of AC and DC technology that are controlled separately).
AC microgrids can present different distribution types: single phase, three phase without neutral and three phase with neutral.
In DC microgrids, there are three main types of distribution: monopolar, bipolar and homopolar. Since there is no reactive power element of a DC system, DC distribution can present some advantages, such as reduction of the reactive power losses and an increased utilization of the capacity of the electrical lines . However, this is perhaps at the cost of technical control and protection design complexity when compared to a traditional AC solution.
Full DC distribution systems have only been used for limited applications so far, such as telecommunication systems, vehicles, ships and traction systems [4]. Hybrid AC/DC microgrids can be seen as a beneficial compromise to facilitate the connection of various AC and DC power sources and load demands with the power system in order to minimize installation and operational costs.
Monitoring and Control
Certain microgrid management strategies gain commercial benefit from maximizing the use of their indigenous renewables or other local generation capacity and selling energy back to the grid at times of suitable price differential. However, they can also independently decouple from the main grid if unexpected outages are caused by severe weather or natural disasters.
For those that are forced to be independent and isolated by virtue of their circumstances and operational needs, for example geographically remote sites or off-limits military camps [1], then the objective is solely to manage the supply and demand resources locally available to best effect.
In any case, a key element required for such a system is a fast, responsive and highly functional techno-economic control management system, to coordinate the dispatch decisions and monitor the technical integrity of the system across timeframes ranging from sub-seconds to a daily or weekly basis.
Given the smaller size of microgrids, their lower inertia, the power electronic characteristics of many renewable supply sources and the limited smoothing of demand spikes across a wider customer base, there can be significant stability control, security and power quality challenges inherent in most applications. It is important that these technical challenges are quantified and understood, that suitable control systems are put in place to manage them, and that their implications for constraint on economic operability are properly captured in any modeling and control framework.
There are many parameters in a microgrid that must be continuously supervised by means of a monitoring system, such as voltage, frequency and power quality. Both AC and DC microgrids can have a mixture of hierarchical and decentralized control systems that carry out such tasks, generally comprising three levels: primary control, secondary control and tertiary control.
- Primary control is often applied in a decentralized manner to require individual elements of the system to be automatically robust to both short-term frequency and voltage disturbances. One can implement microgrid voltage control with the voltage regulated (𝑃𝑉) and unregulated (𝑃𝑄).
- 𝑃𝑄 control is an unregulated bus, which holds the active power 𝑃 and reactive power 𝑄 constant at a bus while the voltage is allowed to vary (unregulated) over a limited range.
- 𝑃𝑉 control is a regulated bus, which holds the active power 𝑃 and the bus voltage 𝑉 constant. Since the voltage is held constant, it is considered regulated while allowing the reactive power to vary within limits.
- The function of the secondary control is to help the microgrid achieve global controllability by restoring the frequency and amplitude deviations produced by the primary control. Often a Microgrid Central Controller (MGCC) is used.
- The MGCC may also include tertiary control. Tertiary control ensures economic optimization, based on energy supply and demand trends over a longer timeframe.
“Mars will be the ultimate microgrid. With no centralized power sources, communities will one day rely on decentralized energy systems.”
- SIEMENS, MICROGRID ON MARS
Where are Microgrids being used?
With a range of flexible configurations possible, microgrids already have applications in university campus demonstration sites, providing backup power for critical infrastructure, facilitating diverse industrial and retail commercial operations, powering island systems and other geographically remote sites. In the more distant future, they could also potentially support life on other planets.
The following sections outline some examples of successful projects that have already been implemented around the world.
The 8,000 acre training base of US Marine Corps Recruit Depot from Parris Island, South Carolina has recently deployed a 10 MW Ameresco microgrid project that features energy efficiency and renewable generation and is designed to withstand storms and earthquakes. The goal of the project is to make such a nationally critical facility capable of operating off-grid for up to 14 days.
Renewable microgrids also offer a route to curb military use of petroleum. The US Department of Defence is one of the largest consumers of petroleum in the world. Navigant Research estimates that the military could save $8-$20 billion over the next 20 years by shifting from a reliance on backup diesel generators to large-scale microgrids.
On Parris Island, the military is expected to save $6.9 million in annual utility and operational costs as a result of the energy system redesign, which includes:
- Replacement of a power plant with 3.5 MW of combined heat and power (CHP) and three diesel backup generators
- 20,000 solar PV modules, installed at carport and ground-mount sites, which provide 5.5 MW. The solar panels provide shelter for more than 500 parking spaces
- Batteries totaling 4 MW / 8 MWh
- Intelligent microgrid controls to assure power supply in the event of central grid outages, and is projected to reduce utility energy demand by 75%, and water consumption by 25%.
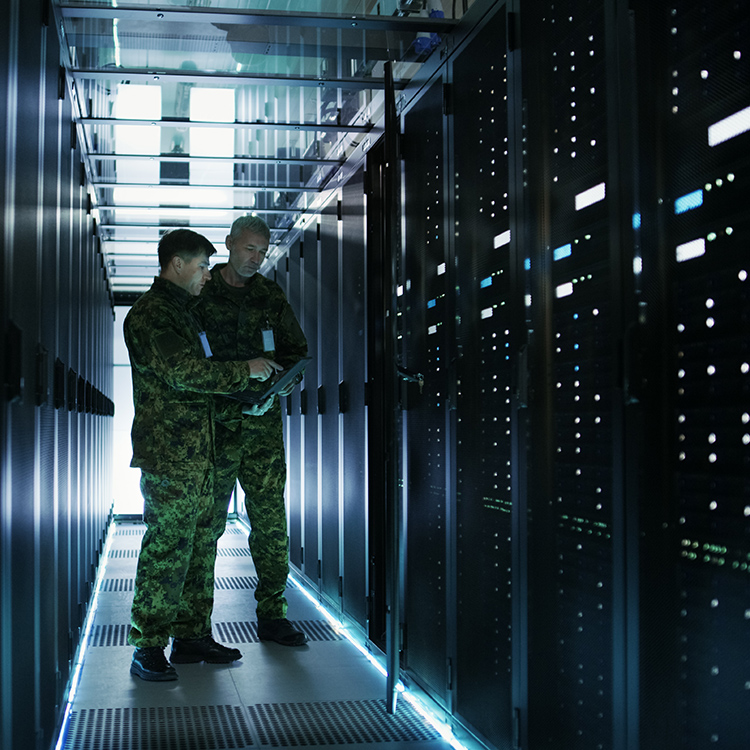
La Graciosa is the unique biosphere reserve from the Canary Islands, Spain. Referred to as "the intelligent energy island", it saves about 35% of total energy after the implementation of a microgrid that includes an estimated 1,500kWh from a photovoltaic system and around 500kWh from a hybrid energy storage system.
Dependent on the energy supply from the Lanzarote island, through a submarine cable, the community of La Graciosa set its objective to ensure local energy autonomy, maximize RES penetration and minimize fossil fuel needs to satisfy the electricity demand from households, commercial and public services on the island [8].
Another crucial motivational example of an island microgrid initiative is the case of Puerto Rico. After Hurricane Maria, which hit the island in 2017 and caused the largest and longest electricity blackout in US History, the island decided to use microgrids as part of the redevelopment and recovery.
The Puerto Rico Energy Bureau has mandated that microgrids be a central part of the island's electricity grid planning. The Bureau has ordered the country's power authority (PREPA) to "directly incorporate the promotion of microgrid resources into all of its transmission, distribution, and resource planning," noting that microgrids "form a critical part of the resiliency solutions envisioned."
The plan calls for Puerto Rico to have renewables make up 40% of the electric supply by 2025 and 100% by 2050.
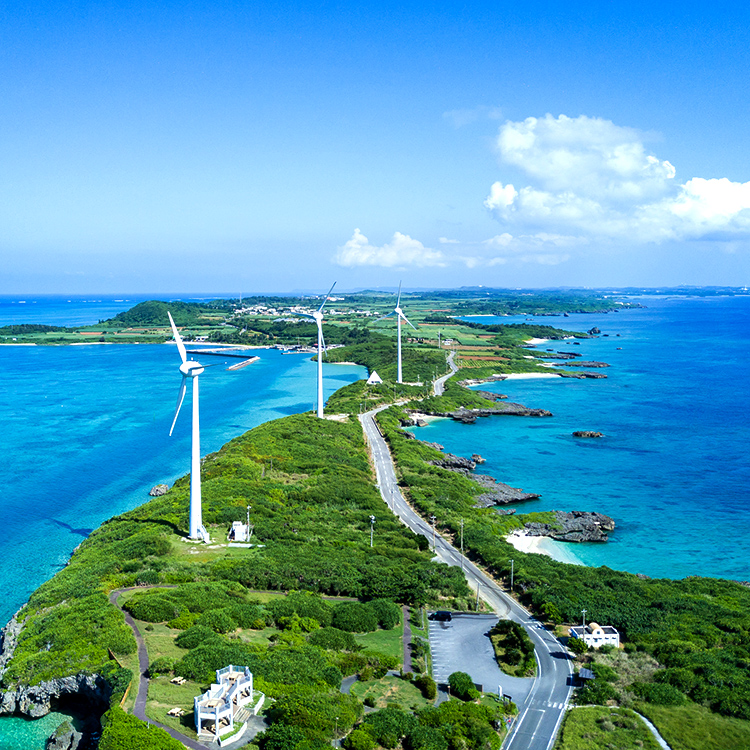
Located at Gold Fields’ Agnew Mine in Northern Goldfields, Western Australia, a microgrid implemented by global energy group EDL combines 18 MW of wind power with 16 MW of baseload natural gas generation, 4 MW of solar and 13 MW/4 MWh-based energy storage.
The hybrid microgrid comes with expectations of reducing energy costs, enhancing energy reliability and resiliency, and reducing the mining facility’s carbon and overall environmental footprint.
Under construction, the hybrid microgrid is expected to meet on average 55-65% of the gold mine’s annual energy needs while instantaneously meeting nearly all its energy requirements, some of the time.
A battery system is customized to deliver high rates of power for short durations to meet the mine site’s energy load profile. In addition, it will make use of dynamic load shedding, renewable resource forecasting and load management to maximize renewable energy while maintaining system security, according to the project partners.
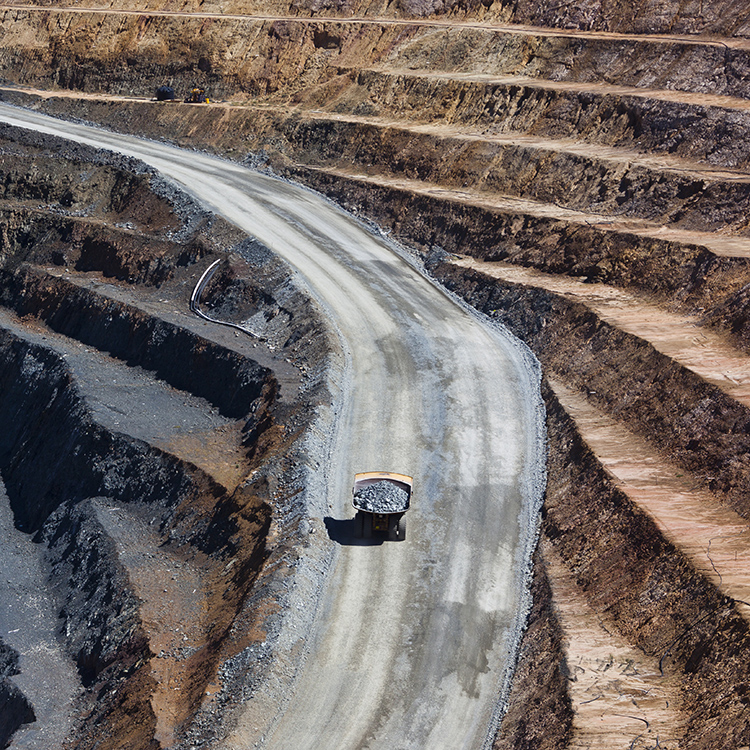
Located in a rural site Themar Al Emarat, in the United Arab Emirates (UAE), micro-grids are helping the Emirate meet its sustainability goals by powering a new hydroponic farm. The Themar Al Emarat hydroponic farm is the largest mushroom farm in the UA, producing nearly six tonnes of mushrooms daily.
Operating the farm in such an arid climate requires significant cooling, maintained by a 5.94 MW off-grid microgrid comprising 23,000 solar photovoltaic cells that will generate as much as 2.7 MW. Another 2.34 MW will come from five, CAT 3412 diesel generator sets. Other core components include bi-directional power electronic inverters and a 286 kWh/250 kW grid stability module incorporated in a CAT battery system.
The project also uses a CAT Master Microgrid Controller, which will autonomously manage the microgrid system, making use of Cat Connect Remote Asset Monitoring for real-time data collection and off-site monitoring of system performance data.
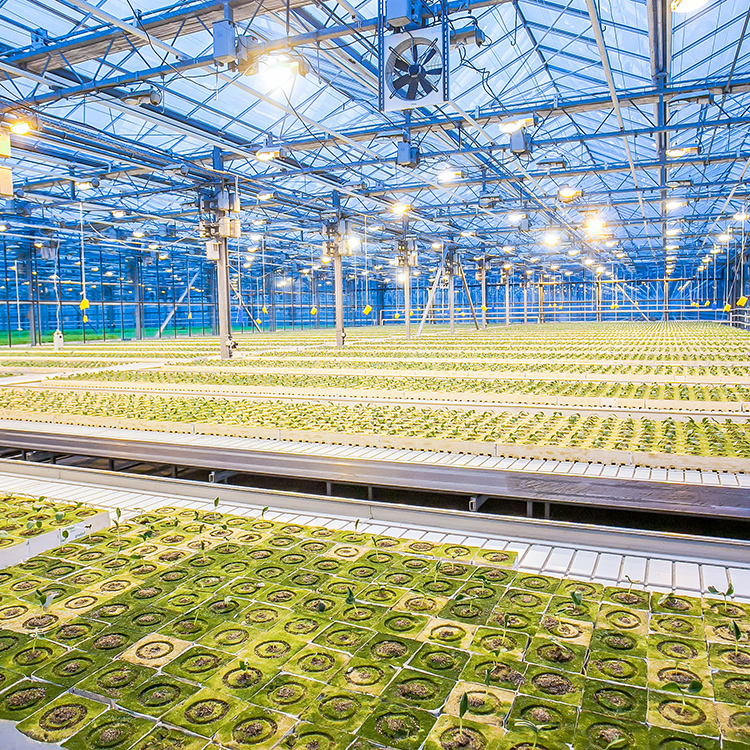
With support from foreign development organizations, Yuma Micro Power has installed 51 off-grid micro power plants in rural Myanmar towns and villages and plans to deliver another 200 by the end of 2019. They combine solar PV, battery energy storage and diesel generators for back-up power, all governed by intelligent microgrid control and management software.
It’s estimated that more than 60% of Myanmar’s population lacks access to modern electricity services. Some 4 million reportedly lack any access to electricity, according to international development agency statistics. Community microgrids co-locate energy generation and consumption, which adds to power system efficiency gains and lowers costs. The decentralized model comes with other advantages that includes enhanced energy reliability, resilience and security and lower greenhouse gas emissions.
For rural electrification the company builds micro power plants that may range anywhere from 15 kW — the size for a completed rural community pilot project — to an anticipated 500 kW of generation capacity.
In addition to its 2019 goal of rolling out 200 microgrids, Yoma Micro Power has yet more ambitious, longer term goals. It’s aiming to deploy some 2,000-community solar microgrids across Myanmar by 2022. It is anticipated that Myanmar can leapfrog a generation of centralized power and move directly to a low-carbon decentralized system.
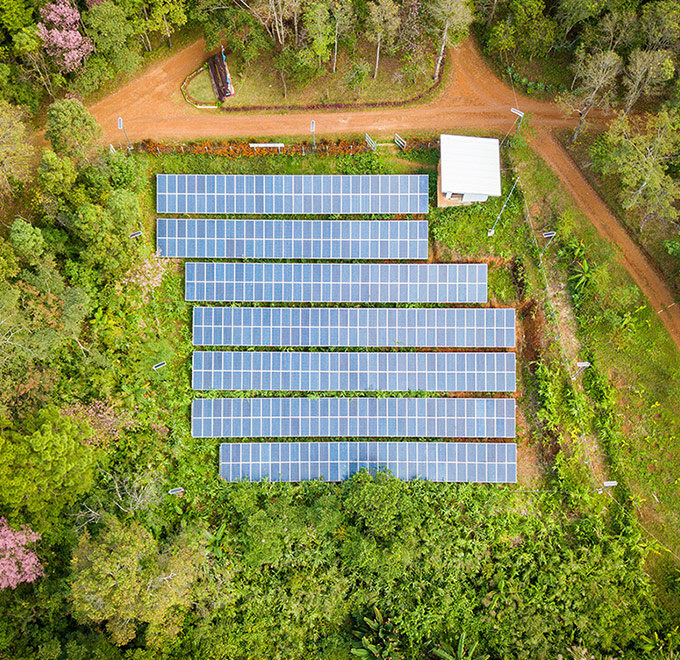
Colleges and universities were among the first institutions to embrace microgrids. Since many campuses have a physical plant to supply steam for heating, their large and well-defined load makes them ideal for installing microgrids. Instead of burning fuel to make steam, universities can benefit from integrating their entire system to cover energy and heating demands. Across the United States, universities from the University of California at San Diego to Princeton and UT Austin rely on microgrids.
In Nigeria, access to the power grid is lacking, with an estimated 40% of the population connected. The grid suffers from intermittent reliability and high costs when access is available. Along with the World Bank and the African Development Bank, the government launched the Energizing Education program. The Program aims to provide over 600,000 students and teachers at 37 Federal universities and 7 University Teaching Hospitals with uninterrupted power. The universities are also benefiting from new street lighting to improve security and the development of renewable energy training centers on every campus.
To date, five universities have completed the development of their microgrids, with four more projects currently underway.
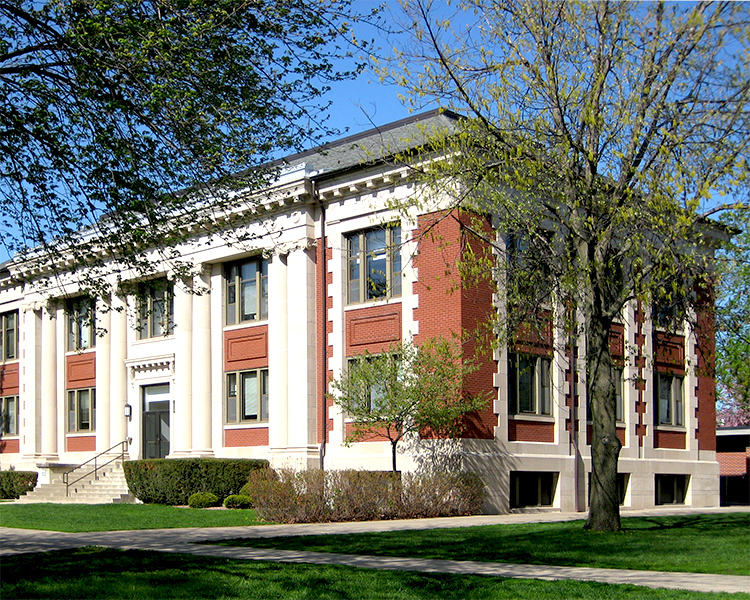
Alaska’s challenges — cold weather and the high cost of delivered energy — are part of the reason the state is a model of innovative microgrid development, with the largest number of microgrids in the US at present, at 122.
Alaska’s innovation began as a response to its lack of centralized power systems. Its electric power infrastructure was created using microgrids.
In fact, in the larger Circumpolar Arctic region, 1.5 million people are served by microgrids in areas that aren’t connected to power grids. The area includes Alaska, Greenland, the Canadian Arctic and large portions of the Russian Arctic.
“Renewable energy has supported the fundamental infrastructure necessary for economic growth and the ability for towns to provide basic services such as public safety and health, lighting, and — most important of all — heat,” said a white paper from Navigant Research.
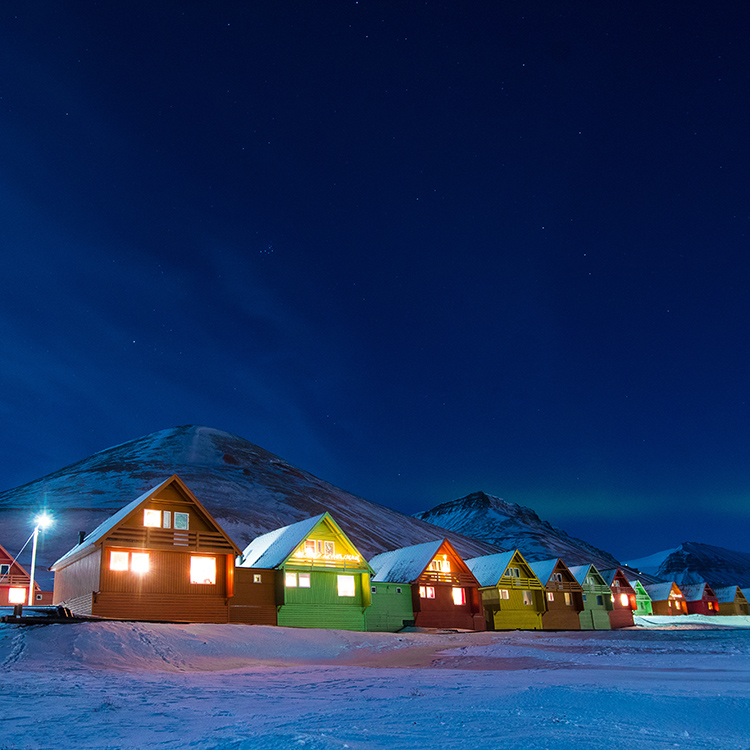
Partners HealthCare, a medical network in New England, is moving forward with plans to install 4.1 MW of Bloom Energy fuel cells this year across its footprint of medical, healthcare, administrative and data center facilities in Massachusetts.
Power outages have not impacted Partners’ ability to operate to date, but Massachusetts suffered more than 100 power grid outages in 2017 lasting a total of 55 hours, according to the Eaton Blackout Tracker.
Another important factor that healthcare institutions are trying to address is ‘decarbonization’. Facilities including solar, CHP/co-generation, energy storage and fuel cells will provide the opportunity through a variety of factors, including sustainability benefits, resiliency and cost savings to powering medical facilities with clean, reliable and affordable energy.
The fuel cells use natural gas and therefore produce less carbon dioxide than utility grid power. They also can run on renewable fuels like biogas and produce virtually no criteria air pollutants (e.g. regulations capping emissions of organic particulate matter).
Adding to the benefits, the fuel-cell systems use little water in normal operation.
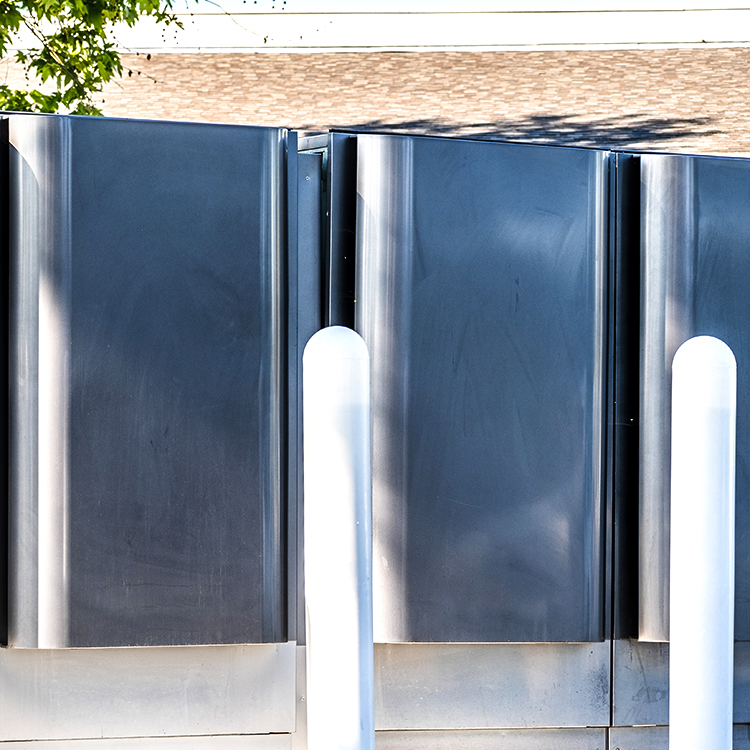
Microgrid Planning with PLEXOS
PLEXOS is the leading unified energy modeling and forecasting software platform designed for modeling your entire micro-grid infrastructure. Its powerful simulation engine analyzes zonal and nodal energy models ranging from long-term investment planning to medium-term operational planning and down to short-term, hourly, and intra-hourly market simulations.
Combining all energy sources from gas, electric, and renewables, PLEXOS allows you to understand the market variations across the entire energy landscape. The result: co-optimized interdependent energy market simulation that enables you to uncover hidden value.
![]() |
Unified SolutionPLEXOS is the only solution available today that unifies market simulations across electric, gas, and water industries. Plus, universal classes allow for optimization beyond the energy market. |
![]() |
Faster and More PowerfulWith powerful new scalability, a modern role-based interface, and improved collaboration features, PLEXOS running in conjunction with PLEXOS Cloud is a revolution in the field of decision analytics. |
![]() |
Simulation Ready DatasetsEliminate market research and spend more time running studies with our simulation ready datasets, curated and analyzed by our data teams. |
Select an option below:
- SHORT-TERM PLANNING APPLICATIONS
- MEDIUM-TERM PLANNING APPLICATIONS
- LONG-TERM PLANNING APPLICATIONS
- ENGINEERING & TECHNICAL APPLICATIONS
- Track the supply and demand balance characteristics with timeframe granularity from 1 second to 1 hour, and timeframe horizon up to 1 week ahead
- Create optimal dispatch decisions for conventional units such as diesel and balance the fluctuations in solar and wind resources
- Model the characteristics of battery storage in detail, with estimation of their cycle-based degradation of power and energy storage capability
- Assess the economic value proposition from demand response and demand shifting from individual buildings or customer groups in the microgrid network
- Use a market model of the external grid source to make optimal decisions for the import/export of energy from grids where PCC capability exists
- Apply stochastic optimization to minimize the ‘expected’ costs or maximize the ‘expected’ profits, based on short-term statistics of wind and solar PV availability
- Track the flow of energy on the microgrid network components so that they are not overloaded and can remain within safe operational limits, and that dispatch can be modified accordingly
- Use the PLEXOS platform’s customization capability to add and model any external EV demand or hydrogen fuel production/supply limits and/or end-use constraints on any demand side response available capability
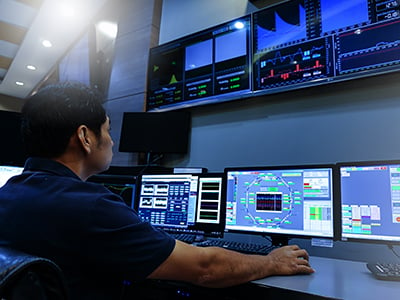
- Develop optimal maintenance schedules for all plant on the microgrid so that repair windows are ideally spaced with respect to customer demand peaks and technical crew constraints, over a 1-year horizon
- Decompose annual budgets for fuel and emissions limits to optimal weekly or monthly breakdowns based on the best available forecasts for supply and demand trends in the microgrid
- Assess reliability levels for customer demand service on the local system
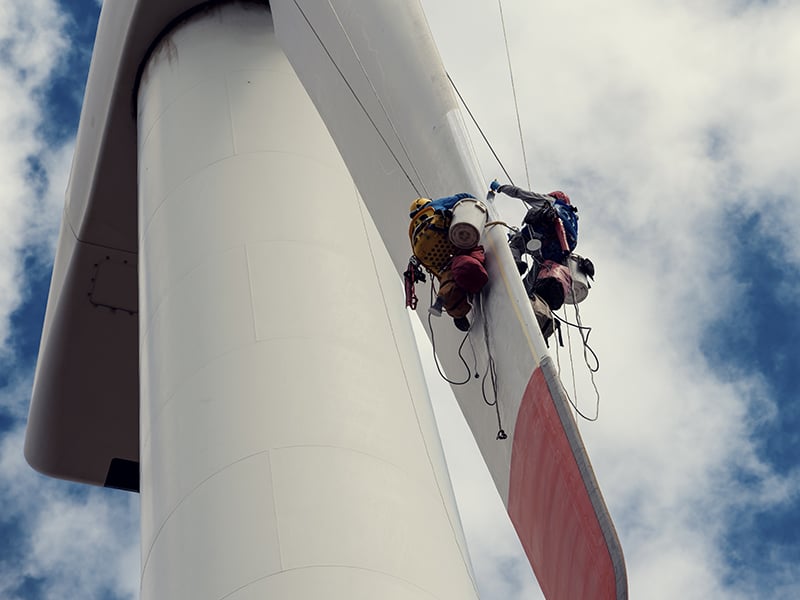
- Apply advanced optimization to decide the least-cost timing, type and amount of investment in new supply resources, as well as the optimal end-of life retirement schedule for existing supplies
- Assess the topology design of the microgrid network and how its constraints may need to be upgraded in the long-term over the next few decades
- Model the capital investment and operational costs (accounting also for depreciation costs), provide respective NPV estimates of the microgrid’s net value at any timeframe horizon
- Plan to meet the microgrid peak and annual energy demand growth forecasts in a reliable manner, capturing the time varying effects of variable renewables, and the energy limits of battery storage
- Consider long-term modeling of uncertainty (e.g. in demand growth, fuel prices, technology costs etc.) in the microgrid development with the use of multiple planning scenarios that can be furthermore assessed employ
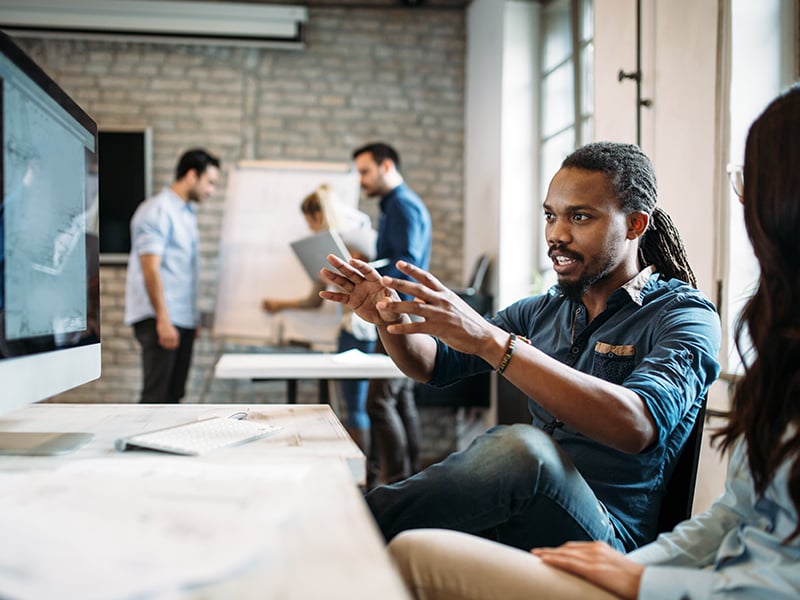
- Model short-term power variation swings from supply and demand on the microgrid, so that frequency stability can be maintained using regulation capability of back-up resources and battery storage
- Use the interface between PLEXOS and engineering software tools such as PSS/E to carry out detailed engineering technical assessments on load flow, voltage & system stability and network constraints, which can be then ‘soft-linked’ back to PLEXOS to accordingly modify optimum dispatch decisions.
- Interface and integrate PLEXOS economic assessments with other tools and energy management systems’ components through a broad range of APIs, e.g. Python, VBA, R and other such tools
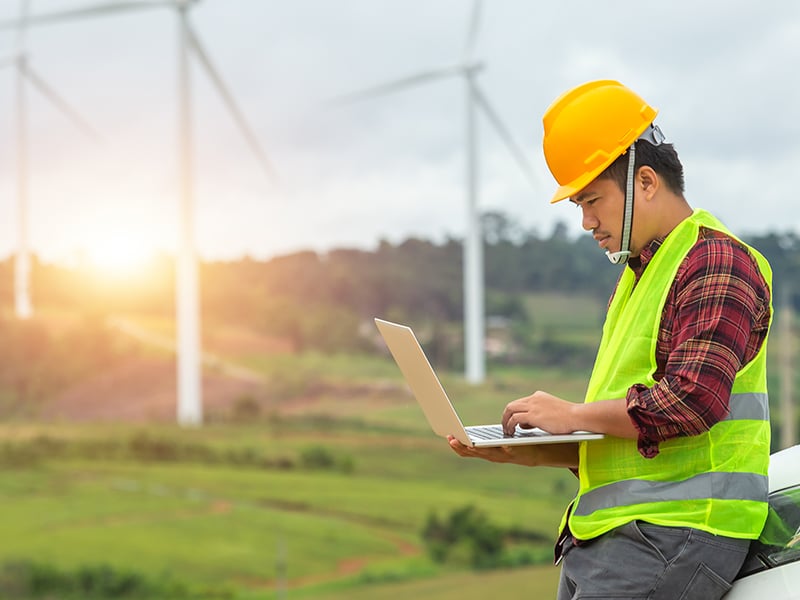
Conclusion
From serving the needs of island communities focusing on decentralized, renewable and local energy, to the increasing requirements for businesses and communities to plan for their own resiliency in the face of supply disruption risks and/or costs, microgrids can offer suitable alternatives to conventional grid solutions in certain applications. While it is unlikely that they will fully eliminate the need for the traditional centralized large scale grids, as the economies of scale and national level energy trends of such will predominate for the foreseeable future, it is assured that smaller scale decentralized grids can play an increasing role to complement the main conventional grid. The integration of microgrids, as functional power supply/demand entities across multiple energy vectors and a range of timeframes, requires the simulation of multiple different scenarios and detailed techno-economic analysis to determine the optimal designs both for a long-term perspective and a near-term operational scheduling perspective. The PLEXOS integrated energy systems simulation platform has the functionality to address the modeling and analytical study of such systems.